Oxygen in Mammalian Cell Culture
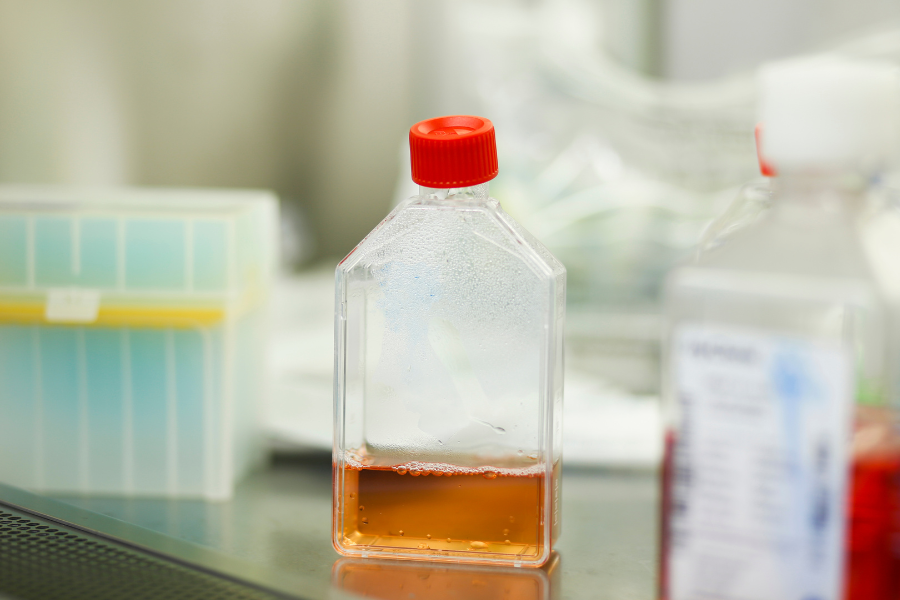
Mammalian cell culture experiments have been instrumental in shaping biomedical research, leading to groundbreaking discoveries about physiological processes and disease mechanisms. These experiments have been critical not only for understanding the origins of diseases but also for developing treatments. Undoubtedly, cell culture remains a cornerstone of both basic and applied biomedical research, routinely used in labs around the world.
However, one often-overlooked factor in cell culture studies is the discrepancy in oxygen levels between the conditions of in vitro experiments and the in vivo environment that cells experience in the body.
Why It’s Crucial to Consider Oxygen Tension
Ambient air has a partial pressure of oxygen (pO₂) of around 160 mmHg, and while the oxygen level is reduced in standard CO₂ incubators for cell culture, it usually remains at about 140 mmHg. This is still significantly higher than the oxygen tensions human cells are typically exposed to. In the body, oxygen levels vary widely depending on the organ and metabolic activity, ranging from 5-100 mmHg. For instance, oxygen tension in the large intestine is between 3-11 mmHg, in the uterus it ranges from 15-19 mmHg, the liver typically has 30-55 mmHg, and the lungs experience the highest oxygen exposure, with alveolar oxygen tensions reaching up to 110 mmHg.
This stark contrast highlights a key point: Standard cell culture conditions expose cells to much higher oxygen levels than they would encounter in vivo, even in tissues that naturally experience elevated oxygen tensions. This difference can influence cellular behavior and may limit the relevance of in vitro findings to actual biological systems.
The Impact of Oxygen Discrepancy on Cell Culture Findings
The higher oxygen levels used in mammalian cell culture do not undermine the many critical discoveries made through these experiments. But it is important to recognize that they can limit our understanding of certain biological processes. These supra-physiological oxygen tensions might reduce the relevance of some in vitro findings when applied to in vivo conditions. Additionally, maintaining mammalian cells under high oxygen concentrations can negatively impact their health, as excessive oxygen levels may hinder their long-term survival and growth. Reducing the oxygen tension to more physiological levels can help improve cell fitness and viability. For example, research has shown that diploid cells can be passaged for significantly longer periods when cultured under lower oxygen conditions (of 20-70 mmHg).
One key phenomenon that illustrates the effect of oxygen on cell culture is the Hayflick limit. This refers to the number of times a normal, somatic (non-reproductive) cell can divide before it stops dividing. While this limit isn’t a fixed number and varies depending on factors such as cell type and culture conditions, it has been demonstrated that the oxygen concentration plays a significant role. A more hypoxic environment can actually extend the number of divisions a cell can undergo, suggesting that oxygen tension is an important factor influencing cell proliferation.
Understanding the Reasons Behind Oxygen’s Impact on Cells
There are several explanations for why oxygen levels influence cellular behavior. One key factor is the formation of reactive oxygen species (ROS). Oxygen can interact with metals and cofactors, leading to the production of ROS. These reactive molecules can cause DNA damage, accelerate cellular aging, and result in other negative consequences. However, it’s important to note that this relationship is not linear—lower oxygen levels do not always equate to less ROS production. Studies show that oxygen concentrations well above the physiological norm can actually increase ROS formation. Additionally, high oxygen levels can directly damage critical cofactors, such as iron-sulfur clusters, which are essential for many biological processes.
Another consideration is that oxygen serves as a substrate in numerous biochemical reactions. When oxygen is present in excess, it can disrupt the balance of these reactions, leading to adverse physiological effects. Oxygen also acts as a signal for regulatory enzymes, which activate signaling pathways that can alter cellular processes. Elevated oxygen levels may trigger different physiological responses through these cascades.
Furthermore, oxygen plays a key role in cellular differentiation. Research shows that lower oxygen concentrations are beneficial for stem cells as they promote enhanced proliferation and reduce harmful effects like DNA damage or unwanted spontaneous differentiation.
Key Impacts of Higher Oxygen Levels on Mammalian Cell Culture Experiments:
- Formation of reactive oxygen species (ROS) leads to DNA damage and other negative effects
- Damage of critical cofactors such as iron-sulfur clusters
- Disrupted flux of biochemical reactions with oxygen as a substrate
- Higher oxygen sensed by the regulatory system may lead to different physiological responses
- Compromised proliferation of stem cells
Implications for Mammalian Cell Research
The use of supra-physiological oxygen concentrations in cell culture is typically not a deliberate choice but rather a matter of convenience. In many cases, cell culture experiments are poorly monitored, and parameters such as oxygen concentration are often neglected entirely.
Proper monitoring and control of oxygen levels in cell culture experiments can more accurately mimic in vivo conditions, which in turn improves the reproducibility and relevance of experimental results. While elevated oxygen levels can have diverse effects on cells, it's also important to note that anoxic conditions (lack of oxygen) can trigger cell death. Therefore, excluding proper gas exchange from cell culture experiments is undesirable.
Finding an experimental setup that allows precise control and adjustment of oxygen concentrations can be challenging. One solution is the use of airtight chambers placed inside standard CO₂ incubators. These chambers can be flushed with a custom gas mixture, but since they are sealed, oxygen levels will change over time as it is metabolized by the cells, and the researcher cannot replenish the gas mix during the experiment. Another option—though significantly more expensive—are tri-gas incubators. These systems allow for the regulation of three gases, typically oxygen, carbon dioxide, and nitrogen, providing more precise control over oxygen levels throughout the experiment. Additionally, dedicated hypoxia workstations, which come with built-in gas controllers, enable long-term culturing of cells under controlled hypoxic conditions.
As a cell culture equipment provider, Biospherix offers such so-called cytocentric (=focusing on the needs of cells) options and many more nuanced solutions for advanced cell culture experiments.
The Importance of Monitoring and Controlling Oxygen Levels
Before attempting to control oxygen levels, it’s essential to closely monitor them. Understanding what is happening within your culture can provide valuable insights into physiological processes that may have previously gone unnoticed. To ensure sterility and accurate measurements, minimally invasive systems such as the DOTS DO Sensor Pills are available, allowing for precise dissolved oxygen measurements in liquid cell culture systems. These systems are easily adaptable to different shake flask sizes and types, making them versatile for a range of applications.
By monitoring and adjusting oxygen concentrations to more physiological levels, mammalian cell culture experiments have the potential to uncover new findings that are more relevant to in vivo conditions. Oxygen is a critical parameter that affects both, cell metabolism and viability. As more experiments begin to incorporate this type of data, we will gain a better understanding of the specific effects oxygen has on cell culture experiments, ultimately allowing us to optimize conditions for greater success in cell culture research.