Scaling Up in Bioprocessing - Bridging the Gap from Lab Bench to Industrial Production
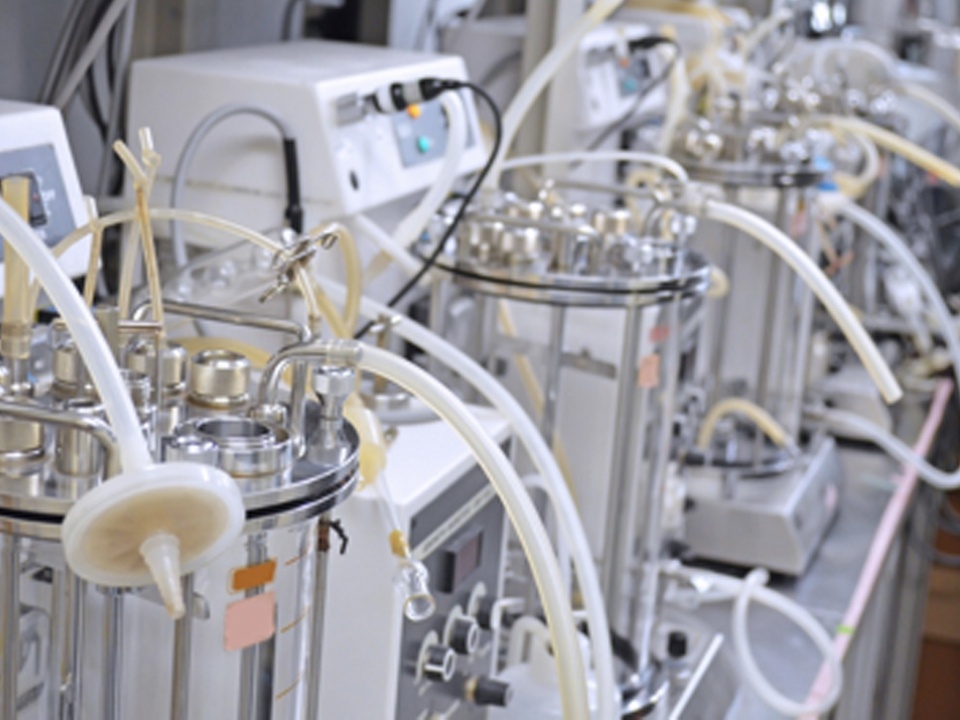
Imagine designing a bioprocess in a lab for months, maybe years. The results look very promising, and you are sure that your process has the potential to make a big difference in the production of a life-saving drug, a sustainable biofuel, or a novel food ingredient. Yet, scaling up is one main barrier to overcome before your work can jump from the lab bench into the market.Scaling up is more than just increasing the size of your bioreactor. It means navigating a series of technical challenges that could suddenly arise between moving from a controlled, small-scale environment into the often severe and uncertain realities of large-scale production. Processes that worked perfectly in a one-liter flask might behave differently in a 10,000-liter tank.
One other point during scale up, is experimental throughput and process information in bioprocessing systems. smaller vessels, like microplates, offer high throughput but provide limited process data. As the vessel size increases, from shake flasks to small scale stirred vessels and finally to fully instrumented bioreactors, the detailed process information improves, but the throughput decreases. Essentially, there’s a balance between quickly running many experiments and gathering in-depth information from more controlled, larger systems for better optimization at the final process
Key Challenges and Concepts
- Oxygen Transfer and Shear Stress
Oxygen Transfer becomes one of the most crucial factors for all aerobic bioprocesses. The larger the bioreactor, the less the surface area available per unit volume. Consequently, enough oxygen cannot uniformly get to the microorganisms. Several times, engineers must install a more elaborate aeration system or introduce a more substantial degree of agitation to ensure proper oxygen transfer. On the other hand, larger agitation can raise the shear stress, harming the cells, particularly the more delicate ones such as mammalian cells.
One such example can be scaling up, where the effective oxygen transport to the cells from a small bioreactor needs to be maintained while increasing its volume. This can be achieved through a change in the speed at which the bioreactor is agitated by devices to increase oxygen distribution.
- Mixing Efficiency
One more critical parameter that becomes more complex with size is mixing. In a small bioreactor, liquid mixing and maintenance of a homogeneous atmosphere are rather easy. In larger bioreactors, it becomes correspondingly difficult to achieve a proper and homogeneous temperature, oxygen, and nutrient distribution throughout its whole volume.
One common metric that engineers investigate to understand the fluid dynamics inside the reactor is the Reynolds number. More macro changes in the fluid flow could result in areas of poor mixing in the reactor. Modifications to the impellers (the shape of blades causing the contents to agitate) or their rotational speed could be done to ensure that all areas of the reactor are mixed properly.
- Heat Transfer
With most biological processes being sensitive to temperature, there is a great need to maintain constant temperatures in a bioreactor. As the quantity of microbial metabolic heat grows, so does the bioreactor volume. This is why larger bioreactors call for increased manner in cooling systems to transfer this heat and prevent possible hotspots from destroying biological activity.
In practice, scaling up means an extreme increase in heat generation, if a small lab-scale bioreactor produces a reasonable amount of heat. Engineers should consider this heightened thermal load by designing cooling systems, often by applying more efficient cooling methods or increasing the surface area for heat exchange.
Pilot Plants and Modeling
Then before scaling up, engineers consider the pilot plants which are intermediate stages that engineers use to test and improve the process before going to full-scale production. These pilot projects facilitate the identification of possible issues and enable modifications in a regulated setting.
In general, scale-up is increasingly powered by mathematical modelling and simulations. With these technologies, engineers could predict the scale-up problems due to, say, oxygen transfer or mixing inefficiencies, and more precisely set up how the process would go when scaled up. For example, simulations of flow and mixing of fluids in a large bioreactor by various simulation programs can be performed and fine-tune the design before building the full-scale system.
Conclusion
If you consider the new tools and techniques, you can turn your shake flasks into low-cost, high-throughput mini bioreactor on a small scale and consider how they help to improve your process before you scale up.
Could digital simulations or real time data from small-scale or pilot scale trials help you for easy handling of sensors and actuators, enabling real-time visualization and analysis of multiple parameters in parallel for optimal bioprocess control and identify potential problems before they arise during scaling up your bioprocess.